Supercomputers Reveal Insights into DNA Repair
Researchers use cutting-edge supercomputers to simulate cellular mechanisms involved in DNA repair, offering new insights into how the body prevents life-threatening diseases caused by DNA damage.
While sunburn and premature aging are common effects of ultraviolet (UV) radiation, tobacco smoke, and other carcinogens, these harmful agents can also cause severe internal damage by breaking apart DNA.
Understanding how the body repairs DNA is key to developing treatments for genetic disorders and diseases like cancer. However, many molecular details of DNA repair remain unclear.
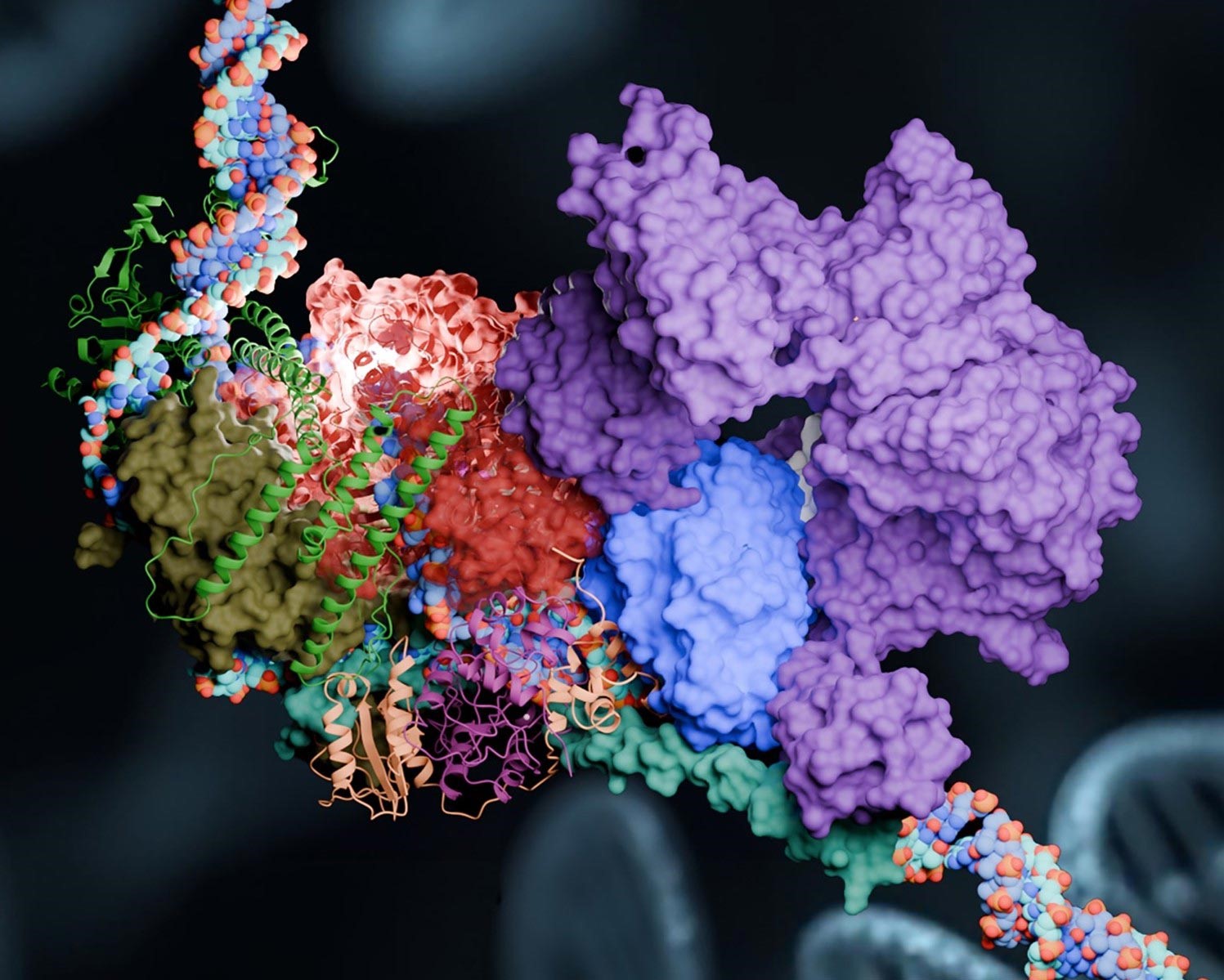
Figure 1. Summit Supercomputer Uncovers Nucleotide Excision Repair Mechanism in DNA Repair
Researchers at Georgia State University are using the Summit supercomputer at the Department of Energy’s Oak Ridge National Laboratory to study nucleotide excision repair (NER), a complex mechanism that detects and removes damaged DNA with remarkable precision [1]. Figure 1 shows Summit Supercomputer Uncovers Nucleotide Excision Repair Mechanism in DNA Repair.
In their latest study published in Nature Communications, researchers developed a detailed computer model of the pre-incision complex (PInC), a crucial component of nucleotide excision repair (NER). PInC regulates DNA repair in the later stages of the NER pathway.
By understanding how PInC functions within the broader repair process, scientists aim to identify new therapeutic targets for cancer and diseases linked to DNA damage and premature aging. Lead investigator Ivaylo Ivanov, a chemistry professor at Georgia State University, emphasized the importance of studying NER, a versatile pathway that fixes various types of DNA damage. However, mutations disrupting this repair system can lead to severe diseases.
Ivanov explained that the effects of genetic mutations vary significantly depending on their location within the repair complexes. “In some cases, mutations lead to extreme sensitivity to UV light and a high risk of cancer. In others, they cause abnormal development and premature aging,” he said. “Why this happens at the molecular level remains unclear. That’s the mystery our computer modeling efforts aim to unravel.”
The Three Stages of DNA Repair
NER unfolds in three distinct stages: recognition, verification, and repair. Each stage requires different groups of proteins to perform specific functions, much like a trauma team has different specialists needed to treat injured patients in the emergency room. In that way, the NER machinery can adapt and change its shape depending on the task at hand.
In the first stage, the NER protein XPC (xeroderma pigmentosum group C) acts like a first responder that locates the site of the damaged DNA, or lesion, and then twists the DNA helix to make the damage accessible. XPC then calls in other repair proteins to help initiate the second stage, called damage verification, or lesion scanning.
Here, the NER protein machinery shifts into its next shape. As XPC steps back, the protein complex called transcription factor IIH, or TFIIH (pronounced T-F-2-H), moves into position. TFIIH further unwinds the section of DNA and scans the newly exposed strand for lesions.
In the final stage of repair, the process is handed over to the molecular “surgeon” — the pre-incision complex (PInC).
With the “patient” stabilized and prepped for surgery, the operation to remove the damaged DNA strand begins. Two enzymes, XPF and XPG (xeroderma pigmentosum groups F and G), position themselves precisely on each side of the lesion and act as molecular scissors to cut out the damaged segment of DNA.
Once the lesion is removed, the gap left behind is filled with newly synthesized DNA, and the DNA backbone is sealed, restoring the damaged DNA to health.
“We aim to understand how the PInC forms after the lesion scanning phase,” Ivanov explained. “How does it control the positioning of the two enzyme subunits that perform the dual incision of the damaged DNA strand? And crucially, is there any communication between the two enzymes? Do they sense each other?”
“These details matter because after the damaged DNA strand is cleaved, the repair process must be completed by filling in the gap,” he added. “Failure to do so could lead to cell death or the introduction of double-stranded breaks, which are extremely harmful to the cell.”
To answer these questions, the researchers needed to solve the structure of the PInC. Understanding protein structure is critical for understanding the behavior and function of protein assemblies. The shapes, sizes, and interactions of proteins determine how they fit together to form larger biomolecular assemblies.
“We integrated the structural model of PInC using data from a variety of biophysical techniques, notably cryo-electron microscopy,” Ivanov said. “But in the end, the computation is what puts everything together.”
Much like assembling a jigsaw puzzle, the PInC model had to be pieced together from known structures of constituent proteins, with many individual components lacking experimental structures. To overcome this, the researchers used a neural network-based model called AlphaFold2 to predict the unknown structures and the interfaces between the proteins that hold PInC together.
Summit's Last Simulations
Ivanov and his team utilized the Summit supercomputer to run extensive molecular dynamics simulations of the PInC complex using NAMD, a code designed for modeling large biomolecular systems. Summit’s immense computational power—capable of 200,000 trillion calculations per second—helped reveal how PInC components move together, forming dynamic substructures crucial for DNA repair.
These insights are significant, as mutations in key NER enzymes, XPF and XPG, are linked to severe genetic disorders such as xeroderma pigmentosum and Cockayne syndrome. The simulations identified critical regions where mutations disrupt NER function, offering a deeper understanding of how these disorders arise.
With Summit retired at the end of 2024, the team now plans to leverage its successor, Frontier, the world’s first exascale supercomputer [2]. Their future research will focus on transcription-coupled NER, a repair pathway essential for maintaining protein synthesis in actively transcribed genes.
References:
- https://www.ornl.gov/news/summit-supercomputer-draws-molecular-blueprint-repairing-damaged-dna
- https://scitechdaily.com/supercomputers-help-unlock-secrets-of-dna-repair/
Cite this article:
Janani R (2025), Supercomputers Reveal Insights into DNA Repair, AnaTechMaz, pp. 364