Researchers Unravel the Mystery of Electrochemical "Catalysis Vs Corrosion"
Engineers from Pitt, Drexel, and Brookhaven have unraveled the mystery surrounding electrochemical ozone production, distinguishing between catalysis and corrosion.
Researchers from the University of Pittsburgh, Drexel University in Philadelphia, and Brookhaven National Laboratory are working together to solve a complex puzzle with the goal of enhancing the sustainability of water disinfection treatments. Scalable electrochemical ozone production (EOP) technologies for disinfecting contaminated water hold promise as potential replacements for current centralized chlorine treatments, applicable in both urban areas and remote communities. Yet, there remains a lack of understanding at the molecular level regarding EOP and how to optimize technologies to ensure efficiency, cost-effectiveness, and sustainability.
Recently, their findings were published in the journal ACS Catalysis. The lead author is Drexel PhD student Rayan Alaufey, with contributions from researchers at Drexel University, including co-PI Maureen Tang, associate professor of chemical and biological engineering, postdoctoral researcher Andrew Lindsay, PhD student Tana Siboonruang, and Ezra Wood, associate professor of chemistry. Additionally, co-PI John A. Keith, associate professor of chemical and petroleum engineering, and graduate student Lingyan Zhao are from Pitt, along with Qin Wu from Brookhaven.
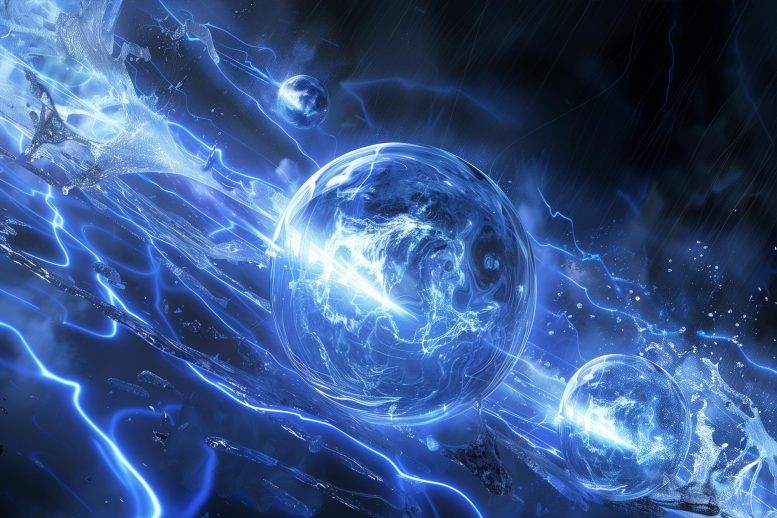
Figure 1. Advancing Electrochemical Ozone Production for Sustainable Water Disinfection: Collaborative Research Insights
The Benefits of Ozone Compared to Chlorine
Figure 1 shows Advancing Electrochemical Ozone Production for Sustainable Water Disinfection: Collaborative Research Insights.[1] "People have relied on chlorine for water treatment since the 19th century, but contemporary understanding suggests it may not always be the optimal choice. For instance, electrochemical ozone production (EOP) can generate ozone, a molecule with similar disinfecting capabilities as chlorine, directly within water. Unlike chlorine, which remains stable in water, ozone naturally decomposes after approximately 20 minutes. This characteristic reduces the likelihood of adverse effects on individuals when consuming tap water, swimming in pools, or undergoing wound cleaning procedures in hospitals," explained Keith, who also serves as the R.K. Mellon Faculty Fellow in Energy at Pitt's Swanson School of Engineering.
"Electrochemical ozone production (EOP) holds promise for sustainable disinfection in certain markets. However, its widespread adoption hinges on the availability of an effective catalyst. Currently, the lack of a suitable EOP catalyst renders the process too costly and energy-intensive for extensive use. My colleagues and I hypothesized that by deciphering the atomic-level mechanisms underlying the functionality of a mediocre EOP catalyst, we could potentially engineer an improved catalyst for EOP."
Exploring the Effectiveness of Catalysts Developed by NATO
Unraveling the puzzle of how EOP catalysts function is pivotal for improving the engineering of one of the most promising and environmentally benign EOP catalysts known to date: nickel- and antimony-doped tin oxide (Ni/Sb–SnO2, or NATO). As Keith highlighted, the challenge lies in understanding the precise role of each atom in NATO towards enhancing EOP. Are the desired ozone-forming catalytic pathways being facilitated, or does ozone result from catalyst decomposition? Future research is essential to enhance the stability of NATO catalysts. Unexpectedly, the researchers found that it likely involves a combination of both mechanisms.
Through a combination of experimental electrochemical analyses, mass spectrometry, and computational quantum chemistry modeling, the researchers constructed an "atomic-scale narrative" elucidating the ozone generation process on NATO electrocatalysts. For the first time, they observed that some of the nickel in NATO may be leaching out of the electrodes due to corrosion. These nickel atoms, now dispersed in the solution near the catalyst, can catalyze chemical reactions that lead to ozone production. "To develop superior electrocatalysts, it's imperative to comprehend both their functional and dysfunctional components. Factors such as metal ion leaching, corrosion, and solution-phase reactions can create an illusion of catalyst performance, masking the actual mechanisms at play," explained Keith.
He emphasized the significance of determining the extent of corrosion and off-catalyst chemical reactions as crucial preliminary steps for researchers seeking to enhance electrochemical ozone production (EOP) and similar electrocatalytic processes. [2] In their closing remarks, they underscored the importance of identifying or disproving such fundamental technological constraints, which are pivotal for the future advancement of EOP and other advanced electrochemical oxidation techniques. "While we understand that electrochemical water treatment is effective on small scales, the key to scaling it globally lies in the discovery of superior catalysts. Our next objective is to identify novel atomic compositions in materials that not only resist corrosion but also facilitate economically and sustainably viable electrochemical ozone production," stated Keith.
References:
- https://scitechdaily.com/scientists-solve-electrochemical-catalysis-vs-corrosion-mystery/
- https://pubs.acs.org/doi/10.1021/acscatal.4c01317
Cite this article:
Janani R (2024), Researchers Unravel the Mystery of Electrochemical "Catalysis Vs Corrosion",Anatechmaz, pp.932